M
Is airborne surface disinfection using hydrogen peroxyde an alternative to formaldehyde?
P. Maris, Anses, Fougères Laboratory (France)
P. Maris (2012). Is airborne surface disinfection using hydrogen peroxyde an alternative to formaldehyde?, EuroReference, No. 6, ER06-12M02. http://www.ansespro.fr/euroreference/numero6/PNB0I0.htm
The spread of disease by airborne contagion had long been suspected and was confirmed around fifty years ago with the discovery that the atmosphere contains a large quantity of inert particles (10 to 20 µm in diameter) that can carry living microorganisms (Noble, 1963). This is dealt with today through the widespread use of airborne surface disinfection processes, particularly in the pharmaceutical, cosmetic and food industries, hospitals, livestock productions, hatchery operations, laboratories, isolators, field stations, and so on. More often than not, the same processes are used in all these different environments, despite the difficulty of transposing application conditions from one field to another. Consequently, users are faced with the constraint of having to implement validation procedures adapted to their specific environment.
Today, airborne surface disinfection processes and the methods for assessing their efficacy are outlined in a French standard (NF T 72-281), the only standard in Europe that addresses the subject. The standard covers two types of process:
- Automatic disinfection processes, conducted without human presence, using droplet or gas dispersion,
- Manual processes, or sprays, conducted with human presence, using a hand, air and/or electric spraying device handled by an operator.
The dawn of the twenty-first century has seen a series of highly significant developments impacting the choices and application conditions of these airborne surface disinfection processes:
- Legislative changes in Europe with regard to chemicals and their impact on human health,
- Health concerns related to the discontinued use of formaldehyde,
- The emergence of decontamination methods in the 1990s, initially within the pharmaceutical industry.
Statutory provisions
- The provisions of the French Government Decree no. 2001-97 of 1 February 2001, establishing special rules for the prevention of carcinogenic, mutagenic, or reprotoxic risks and amending the Labour Code, apply to formaldehyde and any preparation with a higher concentration than 0.1%. The Order of 13 July 2006 (amending the Order of 5 January 1993 listing carcinogenic substances, preparations and processes within the meaning of the second paragraph of Article R.231-56 of the Labour Code) includes formaldehyde. It is classified as Group 1 by the International Agency for Research on Cancer (IARC) and remains a Category 3 carcinogen with risk phase R40 under European Union classification. This French - and possibly future European - position generated renewed interest for airborne surface disinfection, while the search for alternatives to formaldehyde has been made a requirement in France.
- The Biocides Directive 98/8/EC of 16 February 1998 concerning the placing of biocidal products on the market: the objective of introducing this directive is, among others, to assess the harmful effects of biocidal substances and products, as well as their exposure risks, all in relation to their efficacy. Application of this directive is also to be aligned with the European regulation REACH (Registration, Evaluation, Authorisation and Restriction of Chemicals) no. 1907/2006 of 18 December 2006, implemented on 1 June 2007.
The benchmark method for evaluating the potential efficacy of airborne surface disinfection processes prior to their placement on the market is AFNOR NF T 72-281 standard “Procédés de désinfection des surfaces par voie aérienne - Détermination de l'activité bactéricide, fongicide, levuricide et sporicide” (Airborne surface disinfection processes - Determining bactericidal, fungicidal, yeasticidal and sporicidal activity), recently proposed for European standardisation within the framework of CEN TC 216 in early 2011.
- Laboratories must not forget the Order of 16 July 2007 establishing the technical prevention measures, including confinement measures, to be implemented in research, teaching, analysis, anatomy and pathological cytology laboratories, autopsy rooms and industrial and agricultural facilities where workers are liable to exposure to pathogenic biological agents.
Very few chemical substances can currently be used as a gas for chemical sterilisation or applied as a disinfectant. Based on their mode of action, they are grouped with alkylating agents (formaldehyde, ethylene oxide, propylene oxide, beta-propionolactone) and oxidizing agents (hydrogen peroxide, peracetic acid, chlorine dioxide, ozone) (Block, 2001).
Some basic facts
Traditionally, when carrying out the selection process for products for this type of airborne surface disinfection, the following properties need to be taken into consideration (Block, 2001):
- Safety: present no risk to staff. While non-toxic products are preferable, they are unfortunately incompatible with a high level of bactericidal, sporicidal, fungicidal, tuberculocidal and virucidal efficacy. Consequently, preventive measures need to be implemented to avoid exposure and reduce residues to an acceptable level for staff safety.
- Controllability: chemical and/or biological measures need to be implemented to control different stages of the process: while the airborne surface disinfection is being conducted (biological and chemical indicators) and during elimination of the gas following the treatment, so that the premises can be reoccupied safely. These controls also help optimise the application conditions of the treatments.
- Molecular stability: although the substance has to remain stable during the treatment itself, its degradation into harmless sub-products is desirable when being eliminated.
- Compatibility with materials: these substances are naturally highly reactive chemicals, which is why they are so effective, and should be applied under optimal conditions that limit their aggressiveness with respect to what are often numerous and varied materials. What is more, new materials are emerging on a regular basis, giving rise to a need for periodic assessments.
- Rapid action and reoccupation of the premises: depending on the sector in which the processes are applied (food and pharmaceutical industries, livestock productions, hatchery operations, laboratories, field stations, etc.), the work organisation constraints are not the same; the processes chosen and their conditions for use must thus take these constraints into account.
- Permeability and diffusion: the environments to be treated can differ greatly in terms of volume, geometry and ease of access. On one hand, equipment cannot always be dismantled, creating obstacles for the proper diffusion of the product, and on the other, it may be necessary to reach remote corners, genuine niches for microorganisms, which are difficult to access using conventional application techniques like spraying. In these cases, the airflow may need to be stirred to distribute the treatment evenly throughout the facility. In such circumstances, it would also be important to implement carefully distributed biological and/or chemical indicators in order to validate the efficacy of an airborne surface disinfection process.
- Environmental emissions: depending on the diffusion capacity, the degree of decomposition of the products and the type of environment in which the operation is being carried out, special caution must be taken with regard to the risk of emissions outside of the premises.
Formaldehyde fumigation
Paradoxically, while there is a rich and longstanding bibliography on this method of applying formaldehyde, fewer publications have been made in the past twenty years than for hydrogen peroxide and there is less controversy surrounding the process. The long-time use of formaldehyde originates in the empirical knowledge of its efficacy since the late nineteenth century. However, more recently and following the events of 18 September 2001 when letters contaminated with Bacillus anthracis were sent in the post, a number of research avenues have been re-explored (Rogers, 2007).
This active substance acts by alkylating the amino and sulfhydryl groups of proteins and ring nitrogen atoms of purine bases in DNA (Block, 2001). Fumigation can be obtained by heating formaldehyde in an aqueous solution (30-35% m/v) containing methanol to prevent polymerisation, or paraformaldehyde, a formaldehyde polymer in heat-sublimable solid form, above 150°C. Another process, discovered accidentally in 1906, consists of producing formaldehyde gas by mixing potassium permanganate with formalin. Per metre cubed to be treated, 20 g of potassium permanganate is added to 40 ml of 37% formaldehyde solution (Cadirci, 2009; Furuta, 1977).
Despite its widespread use, formaldehyde is a hazard due to the fact that:
- It causes irritation of the skin, eyes and mucous membranes,
- When inhaled in small amounts, it causes coughing and nausea,
- Long-term airborne exposure is linked to nasopharyngeal cancer.
In addition to its toxicity, this process presents further inconveniences: the need for several hours of contact time, residue deposits on surfaces by repolymerisation of the formaldehyde monomer, the need for a high level of humidity (70 to 80% RH), an optimal temperature of 18 to 22°C, high concentrations (4 to 10g/m3) (Hoffmann, 1970) and a long elimination period before reintroducing staff or animals.
To illustrate, here is an example of the different implementation phases of the process:
(1) Ensure the premises are sealed and climate controlled (temperature and humidity),
(2) Vaporisation with formaldehyde or sublimation of paraformaldehyde,
(3) Contact time: 8 to 10 hours to obtain a significant decrease in the bacterial or viral population, under optimal conditions for dosage, temperature and relative humidity,
(4) Neutralise the formaldehyde with ammonia (approximately 2 hours) produced by heating ammonium carbonate (7.5 g/m3) to 120°C (Abraham, 1997),
(5) Eliminate the residual ammonia (approximately 1 hour).
Depending on the type of environment to be treated, phases (4) and (5) are not always performed, in which case it will take anywhere from several to over 24 hours before the formaldehyde has been eliminated and the premises can be reoccupied.
Biological indicators
Abraham (1997) reports on seven years of experience decontaminating laboratories (325 m3) and microbiological safety cabinets (1.7 m3), for a total of 2308 routine decontaminations. Using the sublimation of paraformaldehyde in a 160°C silicone oil bath at a dose of 5 g/m3 (laboratory areas) or the vaporisation of formalin at 8.5 g/m3 (microbiological safety cabinets, MSCs), and the biological indicator Geobacillus stearothermophilus (104 spores on an aluminium strip), he observes the absence of surviving spores in 91% and 81% of cases, for rooms and MSCs respectively. Worthy of note, however, is that in this study, as with many others, viruses had not been taken into account and there are no studies confirming the relevance of choosing this indicator with respect to the objectives.
Munro et al (1999) compare the resistance of three commercial indicators (Bacillus stéarothermophilus or Bacillus subtilis) with Mycobacterium bovis, poliovirus type 1 and Bacillus spp. By sublimating paraformaldehyde (10.6 g/m3) under 57% RH and at 28°C, only one of the three indicators is shown to be representative of the microorganisms tested. In an effort to verify the destruction of Bacillus anthracis, Rogers (2007) concludes that both the commercial indicators tested, Bacillus subtilis and Geobacillus stearothermophilus, are representative. Lastly, Harvey (2011) concludes there is a poor correlation between the viruses of foot-and-mouth disease and swine vesicular disease in the presence of foetal bovine serum, and a commercial indicator, the latter being totally inactivated while the viral titres fell by three log10.
Another phenomenon to bear in mind is the absorption of formaldehyde by various materials. It has been shown that cotton and paper have more affinity with formaldehyde than non-porous surfaces such as glass and stainless steel, so that the resulting efficacy is superior with respect to the same microorganisms. This point should draw attention not only to the nature of the biological indicators, but also the nature of their medium, whether the indicators are prepared in the laboratory itself or received from suppliers. Awareness of this when implementing process validation for airborne surface disinfection will help prevent false negatives through excess formaldehyde and, as a consequence, a false sense of safety (Braswell et al, 1970; Spiner and Hoffmann, 1971).
Alternative processes
Most current research in this area is being done on hydrogen peroxide. This active substance takes the form of a colourless liquid at a concentration of 30% m/m. It is water-soluble and has a boiling point of 106°C. Its degradation in water and oxygen is a strong argument for choosing this disinfectant. As for its mode of action, this substance is an oxidant that generates free radicals, including the hydroxyl radical °OH. This hydroxyl group has a lethal effect on cell membranes, which it destroys by lipid peroxidation, and affects the thiol functions of enzymes and structural proteins (Denyer and Stewart, 1998; Russel and Chopra, 1996).
Modes of action and use: the majority of the technologies available on the market can be divided into what are referred to as “wet” and “dry” technologies:
- Nebulisation (or fogging): the product is sprayed through a nozzle to produce droplets ranging from a few µm to 10 µm in size. The space is thus quickly saturated with this homogenous fog, after which the droplets settle to act on surfaces.
- “Dry vapour”: here, the product is sprayed at high pressure onto a hot plate, once the premises have been dehumidified so that the relative humidity is higher than the point of condensation, thus limiting the effects of corrosion. Prior to the injection of hydrogen peroxide, the relative humidity of the space to be treated is between 20% and 40%.
- “Micro-condensation”: in this case, the relative humidity is the ambient RH of the room, and the high-pressure spray causes a strong increase in the relative humidity and more pronounced condensation.
Scientific literature on the optimal conditions for using hydrogen peroxide reveals controversies (Unger-Bimzcok et al, 2008), as much surrounding the relative humidity and the concentration of hydrogen peroxide as the effect of temperature. Several publications make a direct correlation between the concentration of the product in the air and anti-microbial efficacy (Graham et al, 1992; Hultman et al, 2007). Others show high speeds of decontamination to low concentrations of hydrogen peroxide (Sigwarth and Stärk, 2003; Watling, 2002).
During the high-pressure spraying, while the relative humidity is low, the hydrogen peroxide reaches a higher final concentration of gas when the condensation starts to form on the surfaces. Nevertheless, the condensation is deemed uncontrollable and undesirable since it aggravates corrosion problems and prolongs aeration times. Yet the phenomenon of corrosion is seldom studied in detail. A very recent article (Hassan et al, 2011) discusses a study on the effect of this condensation on a quality of stainless steel, observing that vapour concentrations at 500 and 1000 ppm do not have any effect on the metal, no more than the application of a 35% solution. However, a concentration of 1600 ppm produces significant condensation altering the metal. This phenomenon is explained by the concentrating effect produced during this condensation, bringing the hydrogen peroxide to a level of concentration of 60-70%.
Two very in-depth theoretical and practical studies (Unger-Bimzcok et al, 2008; Watling, 2002) lead in the same direction. These studies assess the monitoring of concentrations of hydrogen peroxide and water in the air, as well as micro-condensation on surfaces. Working with habitual doses of hydrogen peroxide (400, 600 and 800 ppm), they conclude that activity is optimal at either 800 ppm with low humidity or 400 ppm with high humidity. Moreover, they conclude that visible condensation is not necessary to achieve proper inactivation. Too low micro-condensation (1 µg of liquid/mm2) notably diminishes activity. When micro-condensation is between 1 and 2 µg/mm2, there is at 400 ppm a strong decrease in the value of D (time required to divide the population of microorganisms by ten), dropping from 14 min to 4 min. But above micro-condensation of 2.9 µg/mm2, there is no improvement to inactivation.
In the end, based on current knowledge, the different approaches with regard to airborne surface disinfection cycles can be considered with comparable levels of performance, with the observation that sub-visible micro-condensation may be sufficient to obtain strong inactivation, reduce the difficulties of aeration and minimize the risk of corrosion.
The potential applications of these processes are as wide as those of formaldehyde, seeing as it has been mostly the pharmaceutical industry, laboratories and even hospitals that have been more concerned thus far. In poultry farming, particularly for the decontamination of eggs, relatively little research has been done, given the primary method of application is fogging. Depending on the author, the concentration used varies from 1.5% to 5% hydrogen peroxide, without affecting hatchability (Bailey et al, 2001; Cox et al, 1999; Sander and Wilson, 1999; Sheldon and Brake, 1991).
Other studies warrant mention, such as those by Heckert (1997) on the efficacy of a “dry vapour” process against animal viruses. The resistance of viral agents representing different families (Orthomyxoviridae, Reoviridae, Flaviviridae, Paramyxoviridae, Herpesviridae, Picornaviridae) was assessed. Inoculums were deposited on glass or steel strips in the presence of various interfering substances, then exposed to hydrogen peroxide after the high-pressure spraying of a 30% solution (2 g of solution per minute for 30 minutes). These conditions proved to be highly effective, albeit with the following remark: unlike bovine serum as interfering organic matter, the presence of erythrocytes has a notable affect on the efficacy of hydrogen peroxide; one explanation put forth is the catalase and peroxidase found in cells. What is more, this type of process has shown to be of particular interest in the inactivation, as much in vitro as in vivo, of various prion strains (Fichet et al, 2007).
As with what had been developed previously for formaldehyde, the validation of application conditions for the airborne surface disinfection process by hydrogen peroxide is an essential step in the implementation of these treatments. This validation step implies choosing relevant biological indicators, i.e. representative of the biological contaminants found in a variety of soils, at judicious temperatures and levels of humidity. Moreover, the number and positioning of the indicators must be part of a strategy tailored to the volume, geometry, layout and ease of access of the space to be decontaminated.
These difficulties are clearly brought to light in the work of Quanten (2011). In an experiment comparing the two types of process (“wet” and “dry”), the two technologies demonstrated equivalent performance. However, depending on the nature of the contamination, the commercial indicator chosen (Geobacillus stearothermophilus) was effective for bacteria (Escherichia coli and Enterococcus faecalis) but less so for viruses, particularly foot-and-mouth disease and swine fever viruses. Other microorganisms, such as mycobacteria or Staphylococcus aureus, also demonstrated greater resistance than the commercial indicator Geobacillus stearothermophilus during a treatment using hydrogen peroxide at a concentration of 750 ppm (Bennett, 2011).
In conclusion, over and above reasons that fully justify calling the use of formaldehyde into question and the search for alternatives, the use of hydrogen peroxide and formaldehyde for airborne surface disinfection presents many similarities in terms of the necessary command of parameters for successful operations, but also gives rise to a certain number of questions on how to ensure maximum safety.
The processes for airborne surface disinfection using hydrogen peroxide are effective and no real differences have been identified between the two major processes on the market (“wet vapour” and “dry vapour”). Since each environment to be treated is specific, it is important to bear in mind that there is no one formula applicable in all circumstances and that a process validating application conditions is paramount. The environment needs to be climate controlled in advance for temperature and humidity to prevent an overly wide gap between air temperature and surface temperature. In order for the gas to distribute evenly, since the geometry of premises can vary in complexity and ease of access, fans may be required to stir the air. In-depth reflection is required for the choice and number of biological indicators to validate or monitor the performance levels of operations. Those traditionally used (Bacillus atropheus and Geobacillus stearothermophilus) are the benchmark indicators for monitoring moist- or dry-heat sterilisation operations, but are not always relevant for this type of application. Lastly, they do not take into account the wide-ranging diversity of deposits in which these microorganisms can be found.
Is airborne surface disinfection using hydrogen peroxyde an alternative to formaldehyde?

References
Abraham G, Le Blanc Smith PM, Nguyen S. 1997. The effectiveness of gaseous formaldehyde decontamination assessed by biological monitoring. J of the American Biological Safety Association, 2 (1): 30-38
Bailey JS, Cox NA, Berrang ME. 2001. Bactericidal treatment of hatching eggs III: Effect of organic contaminants on efficacy of egg sanitizers. J App. Poult Res, 10: 117-120
Bennett A. 2011. Assessment of the limitations of gaseous disinfectants for containment and animal laboratories. Workshop Formaldehyde Replacement - Epizone. Lelystad, Netherlands. 11-12 January 2011.
Block SS. 2001. Gaseous chemical sterilization. In: Block SS, ed. Disinfection, sterilization, and preservation, 5th ed. Philadelphia: Lippincott Williams and Wilkins: 337-359
Braswell JR, Spiner DR, Hoffmann RK. 1970. Adsorption of formaldehyde by various surfaces during gaseous decontamination. Appl Microbiol, 20 (5): 765-769
Cadirci S. 2009. Disinfection of hatching eggs by formaldehyde fumigation - a review. Archiv für Efflugenkunde,73, 2: 116-123
Chaigneau M. 1977. Stérilisation et désinfection par les gaz. Maisonneuve éd., 344 p.
Cox NA, Berrang ME, Buhr RJ, Bailey JS. 1999. Bactericidal treatment of hatching eggs - II: Use of chemical disinfectants with vacuum to reduce Salmonella. J Appl Poultry Res 8: 321-326
Denyer SP, Stewart GSAB. 1998. Mechanisms of action of disinfectants. International Biodeterioration and Biodegradation, 41: 261-8
Fichet G, Antloga K, Comoy E, Deslys JP, McDonnell G. 2007. Prion inactivation using a new gaseous hydrogen peroxide sterilization process. J of Hospital Infection, 67: 278-286
Furuta K, Sato S. 1977. Studies on the disinfection of hatching eggs. Japan Poultry Sci 14 (1): 27-32
Graham GS, Rickloff JR, Dalmasso JP. 1992. Sterilization of isolators and lyophilisers with hydrogen peroxide in the vapour phase. In: Proceedings of the Parenteral Drug Association, Basel, Switzerland: 32-51
Hassan M, Overfelt RA, Haney RL, Fergus JW. 2011. Hydrogen embrittlement of 4340 steel due to condensation during vaporized hydrogen peroxide treatment. Materials Science and Engineering A, in press.
Harvey Y. 2011. Experience and studies on formaldehyde fumigation at the Institute for Animal health. Workshop Formaldehyde Replacement - Epizone. Lelystad, Netherlands. 11-12 January 2011.
Heckert RH, Best M, Jordan LT, Dulac GC, Eddington DL, Steritt WG. 1997. Efficacy of vaporized hydrogen peroxide against exotic animal viruses. Appl and Envir Microbiol, 63, 10: 3916-3918
Hoffmann RK, Spiner DR. 1970. Effect of relative humidity on the penetrability and sporicidal activity of formaldehyde. Applied Microbiol, 20: 616-619
Hultman C, Hill A, McDonnell G. 2007. The physical chemistry of decontamination with gaseous hydrogen peroxide. Pharm Eng, 27(1):22-32
Munro K., Lanser J, Flower R. 1999. A comparative study of methods to validate formaldehyde decontamination of biological safety cabinets. Appl and Applied and Environ Microbiol, 65, 2: 873-876
Noble WC, Lidwell OM, Kinston D. 1963. The size distribution of airborne particles carrying microorganisms. J Hyg Camb, 1963, 61: 385-391
Quanten K and Koenen F. 2011. Vaporised hydrogen peroxide: a promising alternative for formaldehyde fumigation ? Workshop Formaldehyde Replacement - Epizone. Lelystad, Netherlands. 11-12 January 2011.
Rogers JV. 2007. Formaldehyde gas inactivation of Bacillus anthracis, Bacillus subtilis and Geobacillus stearothermophilus spores on indoor surface materials. J of Applied Microbiol, 103: 1104-1112
Russel AD, Chopra I. 1996. Understanding antibacterial action and resistance, 2nd ed, Elis Horwood, Chichester, UK.
Sander JF, Wilson JL. 1999. Effect of hydrogen peroxide disinfection during incubation of chicken eggs on microbial levels and productivity. Avian Diseases, 43: 227-233
Sheldon BW, Brake J. 1991. Hydrogen peroxide as an alternative hatching egg disinfectant. Poultry Sciences, 70 (5): 1092-1098
Sigwarth V, Stärk A. 2003. Effect of carrier materials on the resistance of spores of bacillus stearothermophilus to gaseous hydrogen peroxide. PDA J Pharm Sci Technol, 57(1):3-11
Spiner DR, Hoffmann RK. 1971. Effect of relative humidity on formaldehyde decontamination. Appl Microbiol, 22 (6): 1138-1140
Torck M. 1985. L'oxyde d'éthylène : agent de stérilisation. Bull Soc Pharm, 1: 103-127
Unger-Bimzcok B, Kottke V, Hertel C, Rauschnabel J. 2008. The influence of humidity, hydrogen peroxide concentration, and condensation on the inactivation of Geobacillus stearothermaphilus spores with hydrogen peroxide vapor. J Pharm Innov, 3: 123-133
Watling D. 2002. Theoretical analysis of the condensation of hydrogen peroxide gas and water vapour as used in surface decontamination. PDA J Pharm Sci Technol, 56(6):291-9

Methods
The use of gases as bactericides dates back to ancient times. Odysseus, upon return to Ithaca after a twenty-year absence, said to his nurse Eurycleia, “Old woman, bring me sulphur and fire in order that I may free the air of its poison and purify this palace.” Then, after he, with the help of his son Telemachus, killed the suitors to his throne who were revelling in the palace, Eurycleia said, “Now all those bodies have been piled up at the courtyard gates, and he's purging his fair home with sulphur. He's kindled a great fire.” (Ian Johnston). During the plague epidemics of the Middle Ages, contaminated houses were not only placed under quarantine, but also treated with burned straw, sulphur, antimony and arsenic. In the late eighteenth century, the first airborne surface disinfection process was described by Guyton-Morveau, who had the idea of creating hydrochloric acid gas through the action of sulphuric acid on sea salt (Chaigneau, 1977). Used widely during the wars of the French Revolution and the Empire to disinfect prisons and hospitals, this aggressive process gradually fell out of use with the emergence of formaldehyde, whose bactericidal properties were discovered by Loew in 1888 and later confirmed by Buchner and Segall. Since then, inventions of “air treatment” diffusion processes have been published on a regular basis.
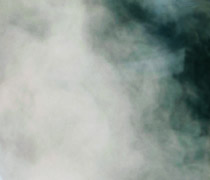